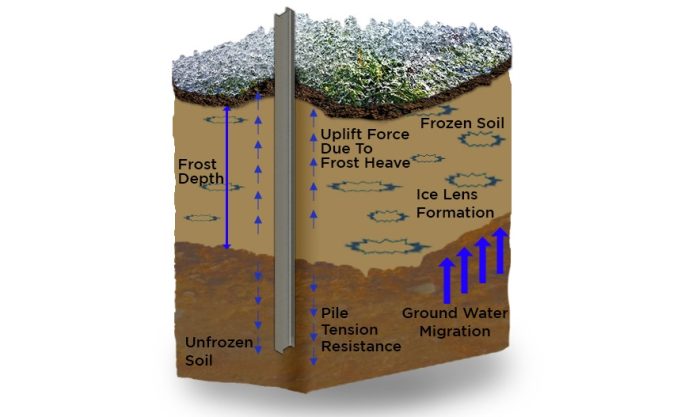
Solar PV modules actually perform better in colder temperatures, but the ground conditions in colder climates can challenge the structural foundation holding up those solar panels. Frost heave is the main point of consideration here.
Frost heave is upward ground movement that occurs as soil freezes. Frost heave occurs because of ice lenses, which form parallel to the surface from water diffused within the soil. As frost penetrates the ground, water is drawn up into the freezing zone, forming layers that force soil particles apart and cause the soil surface to heave.
Check out this post in our archives more on the basics of frost heave and the challenges it presents to ground-mounted solar projects. In this article, we’ll take a look at the cost of not clicking on and reading that post in our archives.
Examples of poor frost heave planning
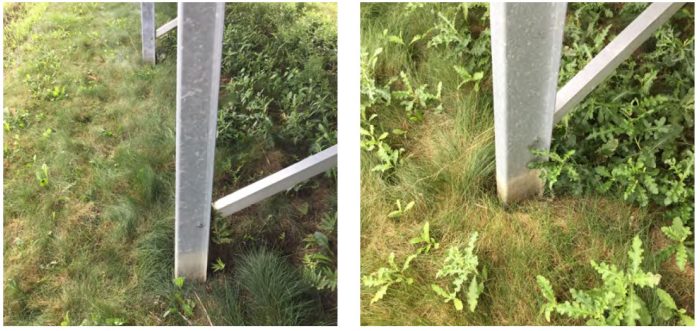
The following examples come courtesy of the Drilling Down on Frost Heave in Utility-Scale PV report from Terrasmart, which includes much more info on frost heave calculations and mitigation strategies.
Poor execution and costly assumptions | In 2015, researchers investigating frost heave at PV farms in Ontario, Canada, found that none of the piles installed at damaged sites had been anchored as they should have been, which is below the actual maximum frost depth. Instead, designers had used lower frost penetration depths because they assumed that snow accumulation would provide some ground cover, reducing frost penetration. Keep reading to see why that was incorrect.
Unfamiliarity with calculating frost depths and adfreeze stress values | So, for PV plants with driven piles, the foundation also can be subject to adfreeze – this is when the frozen soil adheres to the steel surface of the piles. This adfreeze, combined with frost heaving of the soil adjacent to the piles, results in an uplift force known as frost jacking, which lifts the foundation. If you didn’t know that, again, read this piece.
According to a paper published in the American Journal of Civil Engineering and Architecture, investigators discovered that modules installed atop piles deflected snow away from the ground, which meant that there was in fact little to no snow accumulation — and therefore, no cover to mediate frost penetration.
Researchers also found that system designers did not understand that average adfreeze stress values listed in the Canadian Foundation Engineering Manual were low and not as damaging as actual stress values observed in the field. Actual frost depths and adfreeze stress values, they found, often were much greater than those outlined in the manual, prompting them to call for establishing a new factor of safety to be incorporated into future designs.
Ill-equipped local building codes | Solar standards are still nascent / non-existent in many markets, and local building codes are often ill-equipped to accommodate solar, as we discussed last week.
How this relates to frost heave: A 10-MW solar farm had a pile contract set at $1.6 million for materials and installation over the course of six to eight weeks. To stay within budget, the contractor sank pilings only to the minimum depth required by local codes.
By the time spring rolled around, frost heave had displaced nearly every piling. Remediation costs? Nearly $8 million — more than four times the cost of the original contract. The facility’s operational date was also stalled for six months.
Not accounting for varied soil condition on site | In a paper presented to the GEOQuebec 2015 conference, researchers described two private utility-scale PV sites in southwestern Ontario that had been damaged by frost heave during the first winter after they were built.
More than 25,000 steel H-piles were driven to support the two facilities. The 15-foot piles were embedded 11 feet into the ground, leaving four feet of exposure to mount the solar panels above expected snow accumulation levels.
Both sites had varying soils considered among the most susceptible to frost — silt and silty sand, with some silty clay and fine sand reported. Estimated frost depth levels were expected to be between 43 inches and 55 inches.
By the end of the first winter after construction:
- Up to 9 percent of Site A’s piles had moved 1.4 inches — beyond the project’s structural tolerance
- Up to 17 percent of the site A’s piles had moved between 0.8 inches and 1.4 inches
- Site B fared somewhat better, with less than 2 percent of its piles shifting more than 1.4 inches and 9 percent moving between 0.8 inches and 1.4 inches
Site A’s piles shifted most in areas where its groundwater levels were highest, permitting more ice lenses to form closer to the surface.
Several remedial measures have been tried at the Ontario solar farms — including resetting piles with hydraulic pressure, insulating around the piles, and over-excavating to pour concrete bases below the frost depth. The researchers did not spell out costs for remediation, but industry averages would indicate up to four times the original material and installation cost for the foundations.
Frost heave mitigation measures?
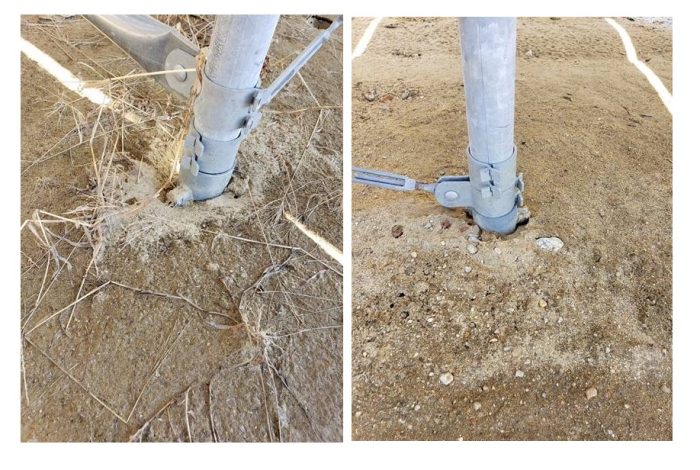
Once engineers know the load that any given foundation might face, here are the options:
- Longer pile: Embedding piles at three times the frost depth to mitigate against frost heave.
- Slick coat: Reducing adfreeze with special coatings to keep piles in the ground,
- Gravel sleeve: Encasing piles in a PVC sleeve or a combination of a sleeve and gravel to prevent frost heave.
- Ballast: Digging below the frost line and pouring a concrete base for each pile may prevent heaving.
- Ground screws: Hollow tube that has multiple threads that lead to a pinpoint.
- Helical: Cylindrical post with a helix attached near the bottom.
Installation and procurement costs of those solutions vary for each of those, of course. And some prove to be more consistently reliable than others – example: driving 18- to 30-foot piles can unwieldy, requiring specialized equipment, and increasing the likelihood of refusal upon striking bedrock, boulders, or cobbles.
Here is how Terrasmart calculates the differences in its report, using 2019 costs:
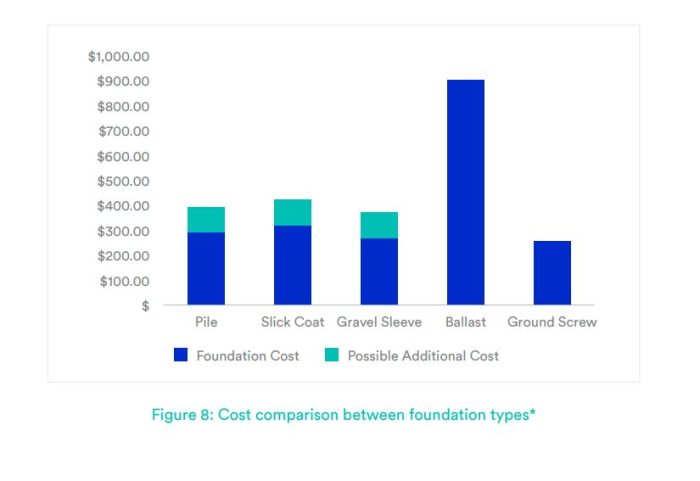
The reasoning underlying that chart:
“None of the options for mitigating frost heave using standard piles is ideal because each measure results in additional costs. Depending on the option selected, the project’s timeline must be extended to allow for special installation requirements. And each potential frost mitigation measure adds to foundation costs. Because the ballast option has been proven to be excessively expensive, it is rarely used. Pile options are more cost effective, but carry some additional cost uncertainty because of the difficulty involved in installing them. This additional cost is represented by the green portion of the chart.”
Note: Terrasmart specializes in ground-screws. But here is the case for ground screws for mitigating frost heave challenges:
“In addition to enabling development of previously inaccessible rocky, steeply sloped sites, ground screws have proven uniquely suited to deep-freezing regions. Because its 34-inch threaded section is embedded below the frost line, a ground screw can resist the upward force of ice lenses while its tip creates a cone of firmly embedded soil that resists frost heave. In addition, the three-inch diameter screw provides less surface area against which frost heave forces can work versus standard driven piles such as W6X9.”
More of the underlying engineering:
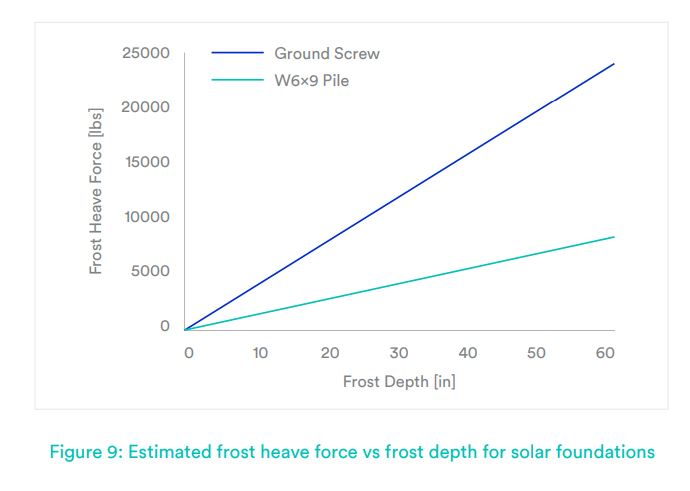
If you’d like to learn more, download the full report right here.
— Solar Builder magazine
Leave a Reply
You must be logged in to post a comment.