The following is a summary of research conducted by Nielson Scientific, NREL, Rensselaer Polytechnic Institute and ARPA-E. For further insight into why this data matters and how it should affect your solar installation business, check out our accompanying webinar this week: The Solar Study: How to Value High Efficiency Solar Panels in Residential Applications.
Almost any product has “premium” versions, whether it’s organic produce, luxury cars or front-row seats on the 50-yard line. Solar panels are no different. Module manufacturers promote their panels based on efficiency, aesthetics, warranty or anything else they feel will differentiate their panel as a premium model and command a higher price. This begs the question, are higher efficiency panels worth the extra cost?
In many cases, the extra panel cost can lead to overall savings at the system level. In fact, our analysis indicates that an effective high-efficiency panel strategy for installers has the potential to result in one or more of the following outcomes:
1. An increase in the average system size,
2. An increase in total MW installed,
3. More sales and/or reduced customer acquisition costs,
4. Higher per system sales,
5. Higher percentage offset of energy usage for customers,
6. A reduction in installed cost per Watt and
7. An increase in profit margin.
Swapping higher-efficiency panels for standard panels provides the most value for installations with high fixed costs and area constraints. Residential systems are most likely to meet these criteria, which is the focus of this article. There are also cases where commercial systems may experience these constraints and see similar benefits from high-efficiency panels. If a system can be increased in size, say 7 kW to 8.5 kW, the fixed costs get spread across more Watts, leading to a lower installed cost per Watt. This same idea is what leads to much lower installation costs for utility-scale systems when compared to residential systems.
In general, unlocking the value of higher kW residential solar power systems is challenging. In many cases, there is not enough usable space on the roof for additional solar panels. This may not be just due to lack of roof area. In some cases, the space available is not ideal due to shading or orientation (i.e., a north facing roof panel). We’ll refer to these cases collectively as “area-constrained.” In other cases, there may be additional space for panels on the roof, but the energy demand from the home does not justify a larger system. We’ll call these cases “usage-constrained.”
For area-constrained systems where it is not possible to install any additional panels but the energy usage of the home is not 100 percent offset by the panels, higher efficiency panels are a great option to increase the system size and reduce system per-Watt-installed costs. In this article, our goal is to provide a better understanding of the economic benefit of larger systems with higher-efficiency panels. The first step toward this goal is a proper understanding of the installation economics of residential solar power systems.
Installed cost model
There are many cost components within a residential solar power system including hardware, labor, overhead and customer acquisition costs, among others. Accurately understanding how these costs change with higher efficiency modules requires an understanding of how each cost component scales as the system size increases and/or the module power rating increases.
The cost components for residential solar power systems fall within these four cost-scaling categories:
• Per Watt variable costs (solar panels that are priced on a per Watt basis).
• Per module variable costs (racking costs, power optimizers, etc.).
• Per system costs (permit costs, jobsite preparation, crew and materials transportation, etc.).
• Per company costs (overhead costs).
The allocation of cost components to these cost-scaling categories can sometimes get complicated, and certain cost components are split between multiple categories. For example, installation labor is split between per system costs and per module costs. Power electronics can be even more complicated, splitting between per system, per module and per Watt, with the split percentage depending heavily on the power electronics architecture (e.g. microinverters, power optimizers or a string inverter). Fig. 1 illustrates the cost components that fit within the four different cost categories.
Changes in each of these categories effects cost depending on if the system is area-constrained or usage-constrained.
For an area-constrained system, if high-efficiency modules are used in place of standard panels to increase the system energy output, the installation itself is almost identical — the same number of modules are installed, and the design and the wiring are essentially the same. In fact, in this case, three out of the four cost categories do not see any increase in cost. The per company costs, per system costs and per module costs all behave as fixed costs in this scenario. The increase in wattage of the system reduces these fixed costs significantly on a per-Watt installed cost basis.
The only cost category in which costs go up is the sales commissions based on the system Watt size and, typically, the module costs per Watt. However, it is not a fundamental rule that per Watt module costs do not increase with higher efficiency. This relationship depends on many factors around production, technology and pricing strategy.
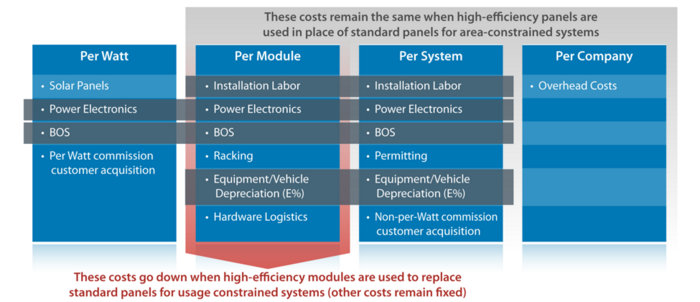
Fig. 1 Residential solar cost components categorized by how that cost component is impacted by module efficiency for area constrained and usage constrained scenarios. Depending on the scenario, costs can increase with the number of Watts or modules in the system or have a fixed system cost at the system or company level. Note that some cost components span multiple cost categories. Also, BOS (Balance of System) costs include wiring, conduit, junction boxes, breakers, switches, hardware logistic costs, and other similar items used in the installation but not specifically called out.
For a usage-constrained system, if high-efficiency modules are used to reduce the module count but maintain the same kW system size, the per module costs would decrease since there are fewer modules (i.e. racking costs would go down since less racking is required with fewer modules), while the other categories would remain the same. This is why the per-module costs shown in Fig. 1 decrease for usage-constrained systems but are not affected by efficiency in an area-constrained scenario.
A key caveat is that the per Watt costs will likely go up due to the higher per Watt module costs for higher efficiency panels (if in fact the higher efficiency panels are more expensive) not because there are more Watts in the system.
Of the two cases, area-constrained systems are more likely to see a reduced installed dollar per Watt system cost and lower levelized cost of energy (LCOE) when using high-efficiency panels. Area-constrained systems with high-efficiency panels will also offset a higher percentage of the homeowner’s energy consumption compared with the same system using standard panels.
However, the total system cost of the area-constrained system will likely go up while the total system cost of the usage-constrained case has the potential to go down. Of course, these results are highly dependent on the price of high-efficiency panels relative to standard panels. In fact, there are many other factors that come into play that determine the financial benefit of high-efficiency panels. Fig. 2 illustrates the high-efficiency panel cost savings variations due to state-specific variations in cost components such as labor rates and taxes for a specific system configuration.
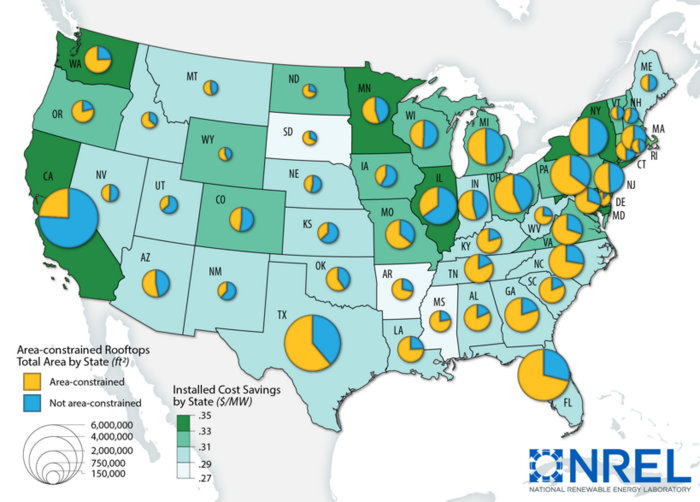
Fig. 2 State level area constrained vs usage constrained systems, market size, and % savings for the case study scenario.
Area- vs. usage-constrained
Since area constrained situations provide the best scenario for unlocking value with high-efficiency panels, an important question is “are area-constrained systems common?” It actually depends significantly on location. Important factors that vary with location include the solar resource, the HVAC load and housing styles (multi-story vs. single-story).
Analysis indicates that across the United States, approximately 60 percent of homes are area-constrained, ranging from around 20 percent in California to over 85 percent in Delaware. The area-constrained vs. usage-constrained ratio for each state is given in Fig. 2. States with a higher percentage of area-constrained homes would tend to benefit more from high-efficiency panels than states with a lower percentage of area-constrained homes.
The percentage of area-constrained homes will increase by electrifying energy consumption that is currently being supplied by fossil fuels. While electrification is slowly progressing on its own, considering electrification at the time of a solar panel installation could provide benefits by allowing the solar system to be sized to offset the additional electric load. This will increase the size of the system either through additional panels (for roofs with space available) or by installing high-efficiency panels.
Increasing solar system size will reduce per Watt costs, as illustrated in Fig. 3. Other important benefits of increasing the system size through expanded electrification include a lower cost of solar energy consumed versus the cost of the alternative energy source from a utility. Local net metering policies, energy rate structures for the location (including possible time of use rates) and any restrictions on state incentives or grid interconnects on system size also influence the value.
New pricing approach?
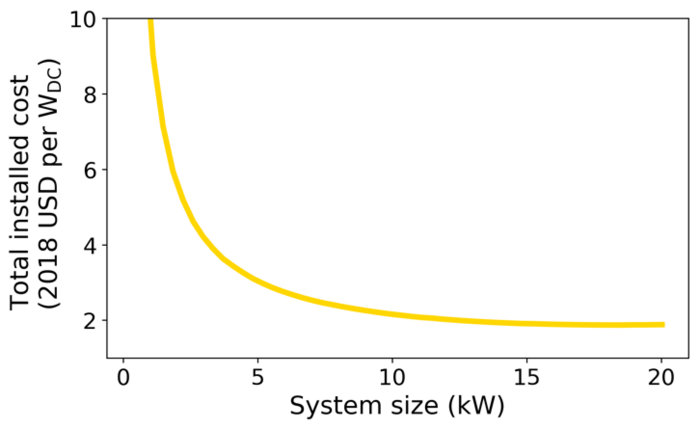
Fig. 3 $/W installed cost vs system size for a system in Oregon using 370W panels purchased at $0.36/W.
Figure 3 also highlights how significantly the installed cost per Watt of small systems grows. For systems in the roughly 3-kW-and-less size range, the cost per Watt becomes so high that it is challenging to make a profit, particularly if the system is priced on a flat per Watt price, as is the industry norm.
The larger solar systems resulting from electrifying homes will also have a lower installed cost per Watt and, if the cost savings are passed on to the customer through a lower system price, a lower LCOE. However, many installers don’t currently pass on install cost savings to homeowners when they price larger sized systems or area-constrained systems upgraded with high-efficiency panels.
In fact, despite the dramatic differences in the dollar per watt installed cost of residential systems of different sizes, residential prices are still typically quoted as a fixed dollar per watt regardless of system size. The industry may want to consider new approaches to pricing residential solar systems. Historically, as installers have found ways to pass on lower installation costs to homeowners, more homeowners have installed solar panels.
Case study
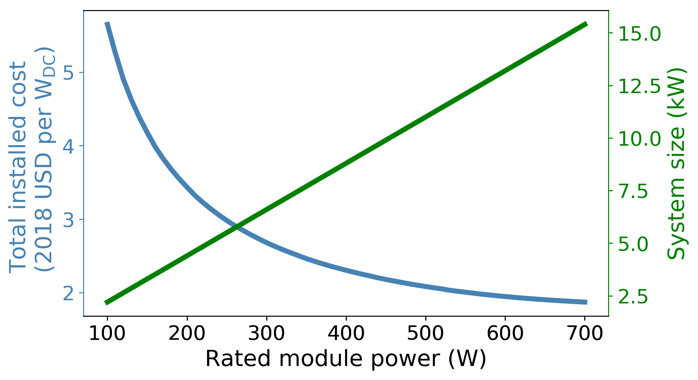
Fig. 4 Installed cost per Watt versus module power rating for the case study system with 24 modules in Oregon.
Let’s consider a case study to help illustrate the benefits of high-efficiency panels for area-constrained residential installations. For this scenario, we will look at a residential system in Oregon that is area-constrained and is limited to 24 panels on the roof.
As the base case, the system will be modeled with standard 60-cell, 300-W solar panels. The high-efficiency case will use 370-W modules. To highlight the per Watt installed cost savings, we will assume that the price of both modules is the same, we’ll assume $0.36/W. It is unlikely that these two modules would actually be the same price, especially with modules so far apart in power rating. However, for the case study, assuming the same price for the modules helps identify what price difference between the modules can be supported while still seeing installed cost savings on a per Watt basis.
By changing the system from a 300-W panel to a 370-W panel, the system increases in size from 6 to 7.6 kW — a significant increase in system size. The total installed cost changes from $19,383 to $20,209, a relatively small increase in overall cost. The installed cost per Watt changes from $2.70/W to $2.28/W, a $0.42/W savings. So, the actual cost of the high-efficiency module could increase by as much as $0.42/W, and the installed cost would still break even on a per Watt basis. There is also a 14.3 percent reduction in LCOE with the high-efficiency panel in this scenario. This assumes all financing and lifetime parameters are the same for each technology: a 9.06 percent nominal discount rate, 2.5 percent inflation, a 25-year lifetime and a 0.5 percent per year degradation rate. The high efficiency panel modeled did have a lower temperature coefficient in this case (-0.35 percent vs. -0.46 percent), which contributed to the lower LCOE of the high-efficiency panel. While higher-efficiency panels often do have lower temperature coefficients, this is not guaranteed.
Understand that the cost benefit of high-efficiency modules changes with the size of the initial system. If the system with standard panels is on the smaller side, the impact of high-efficiency modules will be more significant than if the system with standard panels is on the larger side. The case study system is in the range of a typical residential size system. Table 1 summarizes the case study for Oregon. Figure 2 indicates the cost savings that are possible with this same scenario for all 50 states. Figure 4 illustrates how the installed cost per Watt changes for the case study system in Oregon for different module power ratings.
High-efficiency market and the role of solar installers
Currently, the most commonly installed residential panel is a 60-cell, 300-W mono-PERC module. Sixty-cell equivalent panels with power ratings up to about 375 W are also currently available, enabling roughly a 25 percent increase in system size for area-constrained systems. The highest efficiency panels available make use of cell technologies including n-type silicon, back contacts, heterojunction cell architectures, half-cells and/or shingling of the cells. All currently available high-efficiency panels are based on some type of silicon solar cell.
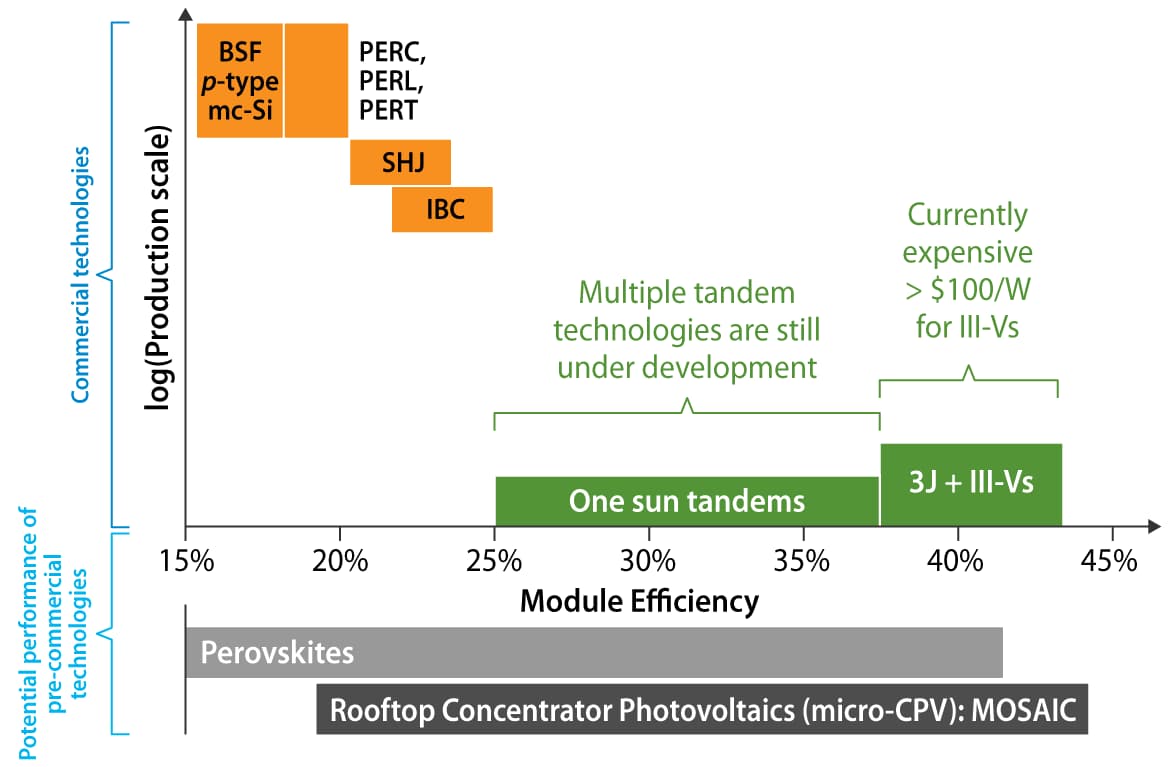
Fig. 5 Chart of potential efficiencies of currently available silicon solar cell technologies and the high-efficiency technologies currently in R&D. The technologies in orange are all c-Si/mc-Si based technologies.
A number of high-efficiency cell and panel technologies are in development that could lead to a 100 percent or more increase in power rating relative to today’s standard 300-W panel. When these technologies are commercialized, 60-cell equivalent panels with power ratings of 600 Watts or more could become available. Technologies with this potential include advanced cells based on III-V semiconductors with or without a silicon cell, as seen in the US Department of Energy’s Advanced Research Projects Agency (ARPA-E) MOSAIC Program technologies or multi-junction III-V GaAs-based or perovskite/silicon tandem cells. Fig. 5 lists these technologies and the potential efficiency levels that they can achieve.
The rate of adoption of higher efficiency technologies by panel manufacturers is influenced by market demand. As demand from installers for higher efficiency panels increases, module and cell manufacturers will more aggressively pursue commercialization of these technologies. Given the potential for high-efficiency panels to reduce installed costs and increase market adoption of solar panels, installers would benefit from exploring how and if these panels could reduce their own costs and increase profitability. Module manufacturers will play a major role in this, as they continue to introduce new PV technology innovations to drive up module efficiency.
Outlook
Although it can be challenging, a data-driven approach for adoption of high-efficiency solar panels can significantly benefit installers and their customers as additional value is unlocked from their solar system. In fact, almost any method that will increase the system size will result in a reduce installed cost per Watt and a reduced LCOE. With 60 percent of residential rooftops in the U.S. being area constrained, the only method available to increase the size of systems for most homes in America is the use of high-efficiency panels. With the anticipated increase in electrification of energy loads with electric vehicles and heat pumps for space and water heating, high-efficiency panels will become even more important for residential installs in the coming years.
Authors: Gregory N. Nielson, Nielson Scientific and Kelsey Horowitz of National Renewable Energy Laboratory; Ashwin Ramdas, National Renewable Energy Laboratory; Benjamin Sigrin, National Renewable Energy Laboratory; Zigurts Majumdar, Rensselaer Polytechnic Institute; Daniel Cunningham, Department of Energy, Advanced Research Projects Agency – Energy (ARPA-E)
— Solar Builder magazine
Leave a Reply
You must be logged in to post a comment.